Last Updated: April 25, 2024
Phospholipases and Phospholipids in Signal Transduction
Phospholipases and phospholipids are involved in the processes of transmitting ligand-receptor induced signals from the plasma membrane to intracellular proteins. The locations at which the various phospholipases hydrolyze phospholipids is described in more detail in the Fatty Acid, Triglyceride, and Phospholipid Synthesis page.
The primary enzymes whose activities are modulated as a consequence of plasma membrane receptor activation are the members of the phospholipase C (PLC) family. Activation of PLC enzymes results in the hydrolysis of membrane phospholipids, primarily phosphatidylinositol-4,5-bisphosphate (PIP2; also designated PtdIns-4,5-P2) leading to an increase in intracellular diacylglycerol (DAG) and inositol-1,4,5-trisphosphate (IP3; also designated Ins-1,4,5-P3). The released IP3 interacts with intracellular membrane receptors that are ion channels leading to an increased release of stored calcium ions (Ca2+). Together, the increased DAG and intracellular free Ca2+ concentrations lead to increased activity of numerous enzymes such as protein kinase C (PKC) and in skeletal and smooth muscle the myosin light-chain kinases (MLCK). Several members of the PKC family are maximally active in the presence of calcium ion (Ca2+) and DAG.
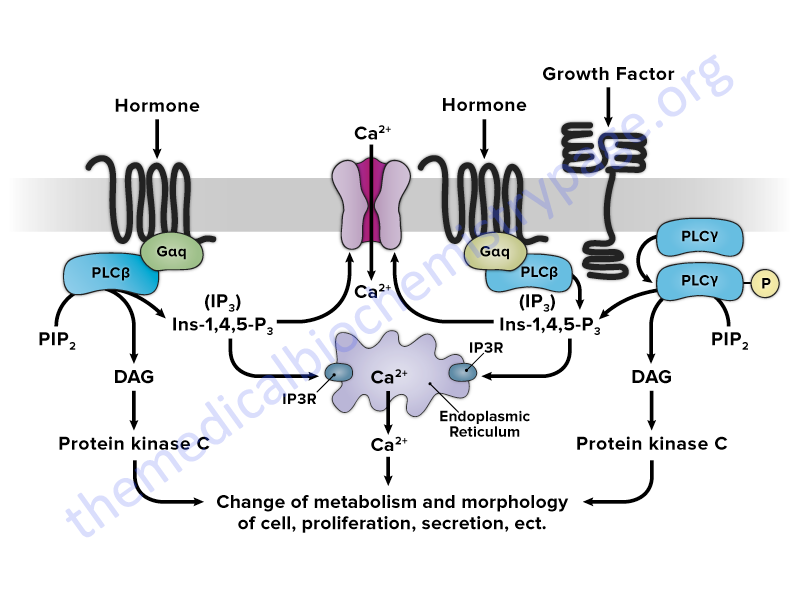
Recent evidence indicates that phospholipases D and A2 (PLD and PLA2) also are involved in the sustained activation of PKC family member enzymes through their hydrolysis of membrane phosphatidylcholine (PC). PLD action on PC leads to the release of phosphatidic acid which in turn is converted to DAG by any one of several members of the phospholipid phosphatase (PLPP) family. PLA2 hydrolyzes PC to yield free fatty acids and lysoPC both of which have been shown to potentiate the DAG mediated activation of PKC enzymes. Of medical significance is the ability of phorbol ester tumor promoters to activate PKC directly. This leads to elevated and unregulated activation of PKC enzymes and the consequent disruption in normal cellular growth and proliferation control leading, ultimately, to neoplasia.
Inositol-1,4,5-Trisphosphate (IP3) Receptors
The inositol-1,4,5-trisphosphate (IP3; Ins-1,4,6-P3) receptors (IP3R) are members of the ligand-gated calcium release channel family that includes the ryanodine receptors of skeletal (RYR1), cardiac (RYR2) muscle, and brain (RYR3). In the case of the IP3R the ligand is inositol-1,4,5-trisphosphate.
In addition to being activated by IP3 binding, the IP3R channel function is regulated by Ca2+ ion at various concentrations. Whereas low cytosolic Ca2+ concentrations facilitate IP3 binding and channel opening, high cytoplasmic Ca2+ concentration inhibits the IP3R.
Humans express three distinct IP3 receptors encoded by the ITPR1, ITPR2, and ITPR3 genes. Each of the IP3 receptors possesses a cytoplasmic N-terminal ligand-binding domain and is comprised of six membrane-spanning helices that form the core of the ion pore. Functional IP3R result from both homotetrameric and heterotetrameric assembly of the large (~2,700 amino acids) IP3R proteins.
The ITPR1 gene is located on chromosome 3p26.1 and is composed of 63 exons that generate three alternatively spliced mRNAs encoding three distinct isoforms of the receptor. ITPR1 isoform 1 is a 2710 amino acid protein, isoform 2 is a 2695 amino acid protein, and isoform 3 is a 2743 amino acid protein.
The ITPR2 gene is located on chromosome 12p11 and is composed of 60 exons that encode a 2701 amino acid protein.
The ITPR3 gene is located on chromosome 6p21 and is composed of 61 exons that encode a 2671 amino acid protein.
Diacylglycerol Kinases (DGK)
Diacylglycerol kinases (DGK) are important mediators of signal transduction pathways involving the phosphoinositides. This family of enzymes catalyzes the phosphorylation of diacylglycerols (DAG) forming phosphatidic acid (PA). The DGK are involved in DAG and PA metabolism in the plasma membrane, the endoplasmic reticulum (ER) membrane, the nucleus, and in the cytoskeleton.
Although DGK are major regulators of the pool of PA they do not represent the sole mechanism for PA synthesis. The sequential acylation of glycerol-3-phosphate and lysophosphatidic acid represents the de novo pathway for PA synthesis.
Humans express 10 genes that encode DGK identified as DGKA, DGKB, DGKD, DGKE, DGKG, DGKH, DGKI, DGKK, DGKQ, and DGKZ. These 10 genes encode diacylglycerol kinase α (alpha), β (beta), δ (delta), ε (epsilon), γ (gamma), η (eta), ι (iota), κ (kappa), θ (theta), and ζ (zeta), respectively.
The human DGK isoforms are classified into five types dependent upon the homology of the structural features of the enzymes. The DGK have at least two cysteine-rich C1 domains (C1a and C1b domain) for interacting with DAG and one kinase domain with catalytic and accessory subdomains.
The type I DGK, which includes the alpha, beta, and gamma isoforms, each contain two calcium-binding EF-hand motifs, which enable these isoforms to respond to Ca2+, in addition to the two C1 domains and the catalytic domain.
Type II DGK, which includes the delta, eta, and kappa isoforms, each contain an N-terminal pleckstrin homology (PH) domain that interacts with phosphatidylinositol, a C-terminal sterile α-motif (SAM) domain, and two catalytic domains in addition to the two C1 domains. The SAM domain is composed of around 70 amino acids and is found in numerous proteins. The SAM domain is an interaction domain allowing for homo- and hetero-oligomerization.
Type III DGK comprises a single DGK, DGKε (epsilon). DGKε is the shortest DGK isoform and it contains two C1 domains followed by a catalytic domain. DGKε does not contain a regulatory domain typical of the other DGK family member enzymes. DGKε is also the only membrane-localized DGK enzyme which is conferred by a hydrophobic segment at the N-terminus of the protein. Unlike the other DGK which phosphorylate DAG harboring a variety of different fatty acids on the sn-1 and sn-2 positions of the glycerol backbone, DGK epsilon exhibits a preference for DAG containing stearic acid at the sn-1 position and arachidonic acid at the sn-2 position: 1-stearoyl-2-arachidonoylglycerol. The substrate specificity exhibited by DGKε is conferred by a lipoxygenase-like (LOX-like) motif in the C-terminal portion of the protein. The LOX-like motif is defined as L-X(3−4)-R-X(2)-L-X(4)-G in which X(n) is n residues of any amino acid.
Mutations in the gene (DGKE) encoding DGKε are associated with atypical hemolytic-uremic syndrome (aHUS). Atypical hemolytic-uremic syndrome is characterized by thrombosis in the small vessels of the kidney leading to renal insufficiency and end-stage renal failure early in life.
Type IV DGK, which includes the iota and zeta isoforms, contain two C1 domains followed by a MARCKS homology domain (myristoylated alanine-rich PKC substrate phosphorylation) domain, a catalytic domain, four ankyrin repeats, and a C-terminal PDZ-binding site.
Type V DGK also comprises a single DGK, DGKθ (theta). DGKθ contains a proline and glycine-rich (P/Gr-rich) domain, three C1 domains, a central PH domain, a Ras association domain, and a catalytic domain. The P/G-rich domain is located at the N-terminus of the protein and may represent a domain to which proteins possessing an SH3 domain bind.
Given that DAG and phosphatidic acid (PA) signaling pathways are interconnected, the DGK play a pivotal role in regulation of these processes due to their ability to convert DAG to PA. This reaction effectively attenuates DAG signaling while simultaneously initiating PA signaling.
DAG activates PKC which then interacts with and phosphorylates numerous target substrates, thereby modifying their activities. PA also interacts with numerous proteins modifying their activity. Some of the more than 50 known PA substrates are mTOR (mechanistic target of rapamycin), PP1 (protein phosphatase 1), PKC-ζ(zeta), and PLCγ (specifically PLCγ1).
The activity of the various DGK is regulated by interaction with phospholipids and by specific subcellular localization. In fact, PA represents a positive feedback mechanism as this lipid activates the DGK. The principal phospholipids that regulate DGK activity include phosphatidylcholine (PC), phosphatidylserine (PS), phosphatidylinositol-4,5-bisphosphate [PIP2; PtdIns-4,5-P2], phosphatidylinositol-3,4-bisphosphate [PtsIns-3,4-P2], and PIP3. The type I DGK are dependent on PS and Ca2+ for activity whereas PIP2 inhibits the activity of DGKθ (theta).
Acylglycerol Kinase and Mitochondrial Phospholipids
Acylglycerol kinase (AGK) is a phospholipid kinase that is primarily localized to the mitochondrial inner membrane where it functions in mitochondrial phospholipid homeostasis. Acylglycerol kinase is also identified as multi-substrate lipid kinase (MULK). The identification of AGK came about through an investigation of the underlying cause of Sengers syndrome. Sengers syndrome is a disorder that is characterized by hypertrophic cardiomyopathy, cataracts, and lactic acidosis, with or without skeletal myopathy.
Acylglycerol kinase catalyzes the synthesis of mitochondrial lysophosphatidic acid (LPA) and phosphatidic acid (PA) from monoacylglycerides (MAG) and diacylglycerides (DAG), respectively. The significance of the activity of AGK to overall mitochondrial function is that phospholipids, particularly cardiolipins, in the membranes of these organelles are involved in the regulation of mitochondrial apoptosis and autophagy.
In addition to its kinase activity, AGK serves as a component of the translocase of the mitochondrial inner membrane 22 (TIM22) complex and in this complex it facilitates protein anchoring to the mitochondrial inner membrane. This function of AGK does not involve its kinase function. Additional non-kinase activities have been found to be associated with AGK that involve its localization to the plasma membrane and to the cytosol. When localized in the plasma membrane AGK associates with the phosphoinositide phosphatase, PTEN. When AGK is associated with PTEN the phosphatase activity of PTEN is inhibited which allows the PI3K, AKT/PKB, and mTORC1 signal transduction pathways to remain active.
Acylglycerol kinase is encoded by the AGK gene. The AGK gene is located on chromosome 7q34 and is composed of 18 exons that generate two alternatively spliced mRNAs encoding precursor proteins of 422 amino acids (isoform 1) and 350 amino acids (isoform 2). Expression of the AGK gene is ubiquitous with the highest levels of expression found in the intestines, kidney, and brain.
Phospholipase C (PLC) Family
Members of the phospholipase C (PLC) family play crucial roles in the regulation of signal transduction in a wide array of systems. The members of the PLC family hydrolyze membrane-associated phosphatidylinositol-4,5-bisphosphate (PIP2; PtdIns-4,5-P2) resulting in the generation of two second messengers, inositol-1,4,5-trisphosphate (IP3; Ins-1,4,5-P3) and diacylglycerol (DAG), in response to activation of receptors by hormones, growth factors, and neurotransmitters. Upon its generation, IP3 binds to one of three IP3 receptors (IP3R) present in the membranes of the endoplasmic and sarcoplasmic reticulum (ER and SR) which activate the release of stored Ca2+. The released Ca2+, along with DAG, activates kinases of the protein kinase C (PKC) family.
Thus far, a total of 13 PLC genes have been identified in the human genome. The proteins encoded by these 13 genes have been assigned to six subclasses of enzyme defined on the basis of structure and regulatory activation mechanisms. These six subfamilies are referred to as PLC-beta (PLCβ1–β4), PLC-gamma (PLCγ1 and PLCγ2), PLC-delta (PLCδ1, δ3, and δ4), PLC-epsilon (PLCε), PLC-zeta (PLCζ), and PLC-eta (PLCη1 and PLCη2).
All of the PLC enzymes contain catalytic X and Y domains in addition to subtype-specific domains and domains conserved across multiple members. Each enzyme also contains several regulatory domains, including the C2 domain, EF-hand motif, and the pleckstrin homology (PH) domain.
The C2 domain constitutes a stretch of approximately 130 amino acid residues that is found in proteins that bind phospholipids in either a calcium-dependent or calcium-independent manner. The vast majority of proteins, whose activities are controlled by Ca2+ binding, contain a structural motif referred to as the EF-hand. The EF-hand domain consists of two regions of α-helix linked by a short (usually 12 amino acids) loop region. The PH domain constitutes a stretch of approximately 120 amino acids that is found in numerous proteins that bind phosphoinositides with high affinity and specificity.
Activation of the PLCβ family members occurs via association with GPCR that are coupled to the Gq class of G-protein.
The PLCγ enzymes contain an SH2 domain that allows them to interact with phosphotyrosine residues present on receptors with intrinsic tyrosine kinase activity or with receptor-associated tyrosine kinases.
The PLCε family member is activated by GPCR that are associated with members of the G12/13 G-protein family or by receptors that activate members of the RAS and RHO family of monomeric G-proteins.
Phospholipase A (PLA) Family
The PLA family of lipases consists of the PLA1 and PLA2 subfamilies. The designation of PLA1 or PLA2 relates to the target of the enzyme. PLA1 enzymes catalyze hydrolysis of fatty acids from the sn-1 position of glycerophospholipids generating 2-acyl-lysophospholipids and free fatty acids. PLA2 enzymes catalyze hydrolysis of the sn-2 position of glycerophospholipids releasing free fatty acids and 1-acyl-lysophospholipids.
PLA1 Family
Mammals express several different extracellular enzymes that exhibit PLA1 activity all of which belong to the pancreatic lipase gene family. These enzymes include phosphatidylserine (PS)-specific PLA1 (PS-PLA1), two membrane-associated phosphatidic acid (PA)-selective PLA1 (mPA-PLA1α and mPA-PLA1β), hepatic lipase (HL, encoded by the LIPC gene, also commonly called hepatic triglyceride lipase, HTGL), endothelial cell-derived lipase (EDL, encoded by the LIPG gene), and pancreatic lipase-related protein 2 (PLRP2).
Due to differences in substrate specificities, structural features, and gene organization, PS-PLA1, mPA-PLA1α, and mPA-PLA1β form a subfamily in the pancreatic lipase gene family. In addition, PS-PLA1, mPA-PLA1α, and mPA-PLA1β exhibit only PLA1 activity as well as exhibiting preference for certain phospholipids such as phosphatidylserine (PS) and phosphatidic acid (PA). In contrast, HL, EDL and PLRP2 possess triglyceride-hydrolyzing activity in addition to PLA1 activity.
In addition to the above described enzymes, the pancreatic lipase family of enzymes includes pancreatic lipase (PL) and lipoprotein lipase (LPL) both of which exhibit specificity toward triglycerides.
PS-PLA1 preferentially hydrolyzes phosphatidylserine (PS) hence the naming of this enzyme. The products of PS-PLA1 are a fatty acid and lysophosphatidylserine (lysoPS). Although PS-PLA1 is one enzyme for the generation of lysoPS, removal of the fatty acid at the sn-2 position of PS will also generate a lysoPS. The latter reaction has been shown to be catalyzed by a secreted PLA2 (sPLA2) most likely encoded by the PLA2G2A gene.
LysoPS has been implicated in several biological processes that include suppression of T-cell proliferation, activation of mast cells, induction of fibroblast and glioma cell chemotaxis, and the promotion of neurite outgrowth. LysoPS has been shown to bind to and activate at least three GPCR identified as GPR34, P2Y10, and GPR174. It has been proposed that these three receptors be renamed to LPS1, LPS2, and LPS3, respectively. Activation of GPR34 (LPS1) is greatest for lysoPS containing a fatty acid at the sn-2 position, a 1-acyl lysoPS.
PLA2 Family
The mammalian genome contains more than 30 genes encoding PLA2 and PLA2-related enzymes. All of these genes are subdivided into several classes that includes low-molecular-weight secreted PLA2 (sPLA2), Ca2+-dependent cytosolic PLA2 (cPLA2), Ca2+-independent PLA2 (iPLA2), platelet-activating factor acetylhydrolases (PAF-AH), lysosomal PLA2, and an adipose tissue enriched member, phospholipase A and acyltransferase 3 (originally designated AdPLA). The intracellular cPLA2 and iPLA2 families and the extracellular sPLA2 family are recognized as the most significant PLA2 enzyme families.
The sPLA2 family contains ten identified enzymes. The sPLA2 family affects various biological events by modulating the extracellular phospholipid environment.
The cPLA2 family contains six members. The cPLA2 enzymes all contain an N-terminal domain that is required for calcium-binding and association with membranes. cPLA2α (the prototypic cPLA2) plays a major role in the initiation of arachidonic acid metabolism.
The iPLA2 family is composed of nine enzymes and is also referred to as the patatin-like phospholipase domain-containing lipase (PNPLA) family. The patatin domain was originally discovered in lipid hydrolases of certain plants and named after the most abundant protein of the potato tuber, patatin. One member of this PNPLA family is adipose triglyceride lipase (ATGL, less commonly PNPLA2) which is described in detail in the Lipolysis and Fatty Acid Oxidation page.
The PAF-AH family contains four members each of which has substrate specificity for PAF and/or oxidized phospholipids.
Table of PLA2 Family Members
Family | Classification | Gene Symbol | Other names | Comments |
sPLA2 | IB | PLA2G1B | pancreatic PLA2 | protects membranes from oxidative damage; SNP in gene associated with increased central adiposity |
sPLA2 | IIA | PLA2G2A | sPLA2 | promotes stimulus-induced arachidonic acid release via the heparan-sulfate proteoglycan (HSPG) pathway; evidence indicates that PLA2GA2 may play a critical role in suppression of the progression and/or metastasis of gastric cancers |
sPLA2 | IIC | PLA2G2C | may be a pseudogene | |
sPLA2 | IID | PLA2G2D | originally called secretory-type PLA, stroma-associated homolog (SPLASH); substrate preferences are phosphatidylglycerol (PG) and phosphatidylethanolamine (PE); promotes stimulus-induced arachidonic acid release via the heparan-sulfate proteoglycan (HSPG) pathway | |
sPLA2 | IIE | PLA2G2E | promotes stimulus-induced arachidonic acid release via the heparan-sulfate proteoglycan (HSPG) pathway that is also used by PLA2G2A and PLA2G2D | |
sPLA2 | IIF | PLA2G2F | preferentially releases arachidonic acid, expression stimulated in inflammatory conditions | |
sPLA2 | III | PLA2G3 | substrate preference is phosphatidylglycerol (PG); highly expressed in microvascular endothelium in response to inflammation, ischemia, and cancer; promotes spontaneous arachidonic acid release and prostaglandin production | |
sPLA2 | V | PLA2G5 | predominantly localized to the inner and outer plexiform layers of the eye; mutations in gene result in fleck retina, familial benign (FRFB) | |
sPLA2 | X | PLA2G10 | encodes two mRNAs, smaller one expressed in pancreas, lung, and colon; larger one expressed in spleen, thymus, and peripheral blood leukocytes | |
sPLA2 | XIIA | PLA2G12A | acts as a neural inducer in ectoderm by blocking signaling induced by bone morphogenetic protein (BMP) | |
sPLA2 | XIIB | PLA2G12B | is catalytically inactive due to an amino acid change in its active site and has altered phospholipid-binding properties | |
cPLA2 | IVA | PLA2G4A | cPLA2α | is a negative regulator of growth, specifically of striated muscle |
cPLA2 | IVB | PLA2G4B | cPLA2β | member of the cytosolic PLA2 family |
cPLA2 | IVC | PLA2G4C | cPLA2γ | member of the cytosolic PLA2 family; is membrane-associated and dependent on Ca2+ for activity |
cPLA2 | IVD | PLA2G4D | cPLA2δ | exhibits specificity for linoleic acid; expression is specifically upregulated in the upper epidermis of inflammatory skin |
cPLA2 | IVE | PLA2G4E | cPLA2ε | Ca2+-dependent N-acyltransferase involved in the biosynthesis of N-acyl ethanolamines (NAE) in the brain; transfers the sn-1 fatty acyl chain of phosphatidylcholine to the amine group of phosphatidylethanolamine generating N-acyl phosphatidylethanolamine (NAPE) which are the precursors of bioactive NAE |
cPLA2 | IVF | PLA2G4F | cPLA2ζ | selectively hydrolyzes arachidonic acid from the sn-2 position of membrane phospholipids providing the precursor for eicosanoid biosynthesis |
iPLA2 | VIA | PLA2G6 | iPLA2β, PNPLA9 | mutations in gene are associated with neurodegeneration with brain iron accumulation 2B (NBIA2B) |
iPLA2 | VIB | PNPLA8 | iPLA2γ, PNPLA-γ | mutations in this gene associated with mitochondrial myopathy with lactic acidosis |
iPLA2 | PNPLA6 | PNPLA6 | iPLA2δ, neuropathy target esterase (NTE) | deacetylates intracellular phosphatidylcholine to produce glycerophosphocholine; important in maintaining axonal integrity |
iPLA2 | PNPLA7 | PNPLA7 | NTE-like 1 (NTEL1) | a lysophospholipase that preferentially deacylates unsaturated lysophosphatidylcholine (lysoPC); implicated in regulation of adipocyte differentiation |
iPLA2 | PNPLA3 | PNPLA3 | iPLA2ε, adiponutrin (ADPN) | expression primarily in liver and adipose tissue; expression is increased dramatically during induction of adipocyte differentiation; expression also induced by glucose; preferential triglyceride lipase activity; transfers unsaturated fatty acids from triglycerides to phospholipids; polymorphism where Ile 148 is substituted for Met (I148M) is highly correlated with development of NAFLD |
iPLA2 | PNPLA2 | PNPLA2 | iPLA2ζ, ATGL | major regulator of adipose tissue triglyceride hydrolysis; catalyzes the initial step in adipocyte triglyceride hydrolysis; exhibits a strong preference for the hydrolysis of long-chain fatty acid esters at the sn-2 position of the glycerol backbone; functions coordinately with hormone sensitive lipase (LIPE) and diacylglycerol O-acyltransferase 2 (DGAT2) |
iPLA2 | PNPLA4 | PNPLA4 | iPLA2η/GS2 | possesses abundant triglyceride lipase activity; also exhibits acylglycerol transacylase activity |
iPLA2 | PNPLA5 | PNPLA5 | GS2-like | exhibits retinylester hydrolase activity, inhibits transacylation |
iPLA2 | PNPLA1 | PNPLA1 | gene is expressed in epidermal keratinocytes; functions in glycerophospholipid metabolism in the cutaneous barrier; mutations in gene associated with autosomal recessive congenital ichthyosis, ARCI | |
PAF-AH | VIIA | PLA2G7 | plasma PAF-AH Lp-PLA2 | lipoprotein-associated PLA2; secreted by differentiated macrophages but not monocytes |
PAF-AH | VIIB | PAFAH2 | PAF-AH-II | platelet-activating factor (PAF) acetylhydrolase 2; is a single subunit intracellular PAF hydrolyzing enzyme |
PAF-AH | VIIIA, VIIIB | PAFAH1B1 PAFAH1B2 PAFAH1B3 | PAF-AH-Iα1 PAF-AH-Iβ2 PAF-AH-Iγ | platelet-activating factor (PAF) acetylhydrolase isoform 1B; composed of three subunits, α, β, and γ encoded by the indicated genes; in addition to PAF hydrolytic activity the heterotrimeric enzyme is involved in a signal transduction pathway crucial for cerebral development; mutations in the PAFAH1B1 gene associated with Miller-Dieker lissencephaly syndrome (MDLS) |
lysosomal PLA2 | XV | PLA2G15 | LPLA2; lysophospholipase 3 (LYPLA3) | hydrolyzes lysophosphatidylcholine; also has esterase and acetyltransferase activity |
AdPLA | XVI | PLAAT3 | H-Rev107 | possesses PLA1, PLA2, and acyltransferase activities; the O-acyltransferase activity catalyzes transfer of a fatty acyl group from glycerophospholipid to the hydroxyl group of lysophospholipid (lysoPL); the N-acyltransferase activity catalyzes Ca2+-independent transfer of a fatty acyl group from the sn-1 position of phosphatidylcholine and other glycerophospholipids to the primary amine of phosphatidylethanolamine, forming N-acylphosphatidylethanolamine (NAPE), which serves as precursor for N-acylethanolamines (NAE); has a role in tumor suppression |
Phospholipase D (PLD) Family
Humans express two major phospholipase D (PLD) isoforms identified as PLD1 and PLD2. These two enzymes share 50% amino acid sequence homology, the highest of which is in the catalytic domain. Although other PLD isoforms have been characterized, the majority of observable PLD activity can be attributed to these two enzymes. Both PLD1 and PLD2, as well as several splice variants, hydrolyze phospholipids such that the polar head group, attached to the phosphate, is released with the other resulting product from this type of reaction being phosphatidic acid. Both PLD1 and PLD2 are capable of hydrolyzing PC, PE, PS, lysophosphatidylcholine (LPC or lysoPC), and lysophosphatidylserine (LPS or lysoPS). However, these two isoforms are not capable of hydrolyzing PI, PG, or cardiolipin. When the substrate is LPC or LPS the product of PLD action is lysophosphatidic acid (LPA).
In addition to the PLD1 and PLD2 genes, humans express four additional genes that encode proteins of the PLD family. These enzymes are designated PLD3, PLD4, PLD5, and PLD6.
The PLD3 gene encodes a protein that is highly similar to the amino acid sequence of viral PLD enzymes. PLD3 contains an N-terminal type II transmembrane domain that facilitates the insertion of the enzyme into the ER membrane. Although this protein has PLD sequence identity it is has not been conclusively demonstrated that is harbors phospholipid hydrolytic activity.
The protein encoded by the PLD4 gene exhibits specificity for phosphatidylcholine with the products being phosphatidic acid (PA) and choline.
The protein encoded by the PLD5 gene is similar to the PLD3 encoded protein in that it does not have phospholipase activity.
The protein encoded by the PLD6 gene is also known as mitoPLD. The PLD6 protein is associated with mitochondria (hence the mitoPLD nomenclature) where it exhibits substrate specificity for cardiolipin, an abundant mitochondrial lipid. The reaction catalyzed by PLD6 occurs at the surface of the mitochondria and the product, PA, facilitates mitochondrial fusion events.
Lysophospholipase D (lysoPLD) refers to enzyme activities that hydrolyze lysoglycerophospholipids (lysoPL) to produce lysophosphatidic acid (LPA or lysoPA) and an amine. Two major forms of lysoPLD have been characterized, a microsomal and an extracellular form.
The microsomal lysoPLD is encoded by the GDPD1 (glycerophosphodiester phosphodiesterase domain containing 1) gene and exhibits the highest substrate preferences for lyso-platelet activating factor (lysoPAF: 1-O-alkyl-sn-glycero-3-phosphocholine), lysophosphatidylethanolamine (lysoPE), and lysophosphatidylcholine (lysoPC). The GDPD1 encoded enzyme is important in the metabolism of platelet-activating factor (PAF).
The extracellular lysoPLD turned out to be identical to another previously characterized protein defined as a tumor autocrine motility factor and called autotaxin (ATX). ATX possess 5′-nucleotide pyrophosphatase and phosphodiesterase (PDE) activities. Because of this demonstrated enzymatic activity, the approved nomenclature for lysoPLD/ATX is ectonucleotide pyrophosphatase/phosphodiesterase 2 (ENPP2). The ENPP2 gene generates three splice variants that are identified as ATX-alpha (ATX-α, composed of 915 amino acids), ATX-beta (ATX-β, composed of 863 amino acids), and ATX-gamma (ATX-γ, composed of 889 amino acids).
Phosphatidylinositols
Although phosphatidylinositol (PtdIns or PI) represent only a small percentage of total cellular phospholipids, it is a crucial intermediate in numerous signal transduction processes given that it serves as the precursor of several second-messenger molecules. Some of the phosphatidylinositol-derives signaling molecules include phosphatidylinositol-3-phosphate (PtdIns-3-P or PI3P), phosphatidylinositol-4-phosphate (PtdIns-4-P or PI4P), phosphatidylinositol-5-phosphate (PtdIns-5-P or PI5P), phosphatidylinositol-3,4-bisphosphate (PtdIns-3,4-P2; one of the PIP2 molecules), phosphatidylinositol-3,5-bisphosphate (PtdIns-3,5-P2; one of the PIP2 molecules), phosphatidylinositol-4,5-bisphosphate (PtdIns-4,5-P2; the most well studied PIP2), phosphatidylinositol-1,4,5-trisphosphate (PtdIns-1,4,5-P3; one of the PIP3 molecules; the most well studied PIP3), and phosphatidylinositol-3,4,5-trisphosphate (PtdIns-3,4,5-P3; one of the PIP3 molecules).
The formation of the various phosphatidylinositol molecules is the role of various phosphoinositide kinases. The are at least four distinct families of phosphoinositide kinases. Two of these kinase families are identified as phosphatidylinositol 3-kinases (PI3K) and phosphatidylinositol 4-kinases (PI4K). The phosphatidylinositol phosphate kinases (PIPK), of which there are two families, are identified as the phosphatidylinositol-5-phosphate 4-kinases (PIP4K) and phosphatidylinositol-4-phosphate 5-kinases (PIP5K)
Phosphatidylinositol Kinase Family
Phosphatidylinositol 3-kinases, PI3K
Phosphatidylinositol-4,5-bisphosphate (PIP2; also designated PtdIns-4,5-P2) is not only a substrate for the PLC family enzymes, whose actions lead to the release of DAG and IP3, but is also a substrate for a family of kinases that phosphorylate the membrane lipid on the 3-OH on inositol. These kinases are referred to as the phosphatidylinositol-3-kinases, PI3K (also designated PIP3K). The PI3K enzymes represent a family of both receptor-activated and non-receptor-activated phosphatidylinositol phosphate kinases (PIPK).
The PI3K are capable of generating phosphatidylinositol-3-phosphate, PIP (more informatively designated PtdIns-3-P), phosphatidylinositol-3,4-bisphosphate, PIP2 (more informatively designated PtdIns-3,4-P2), and phosphatidylinositol-3,4,5-trisphosphate, PIP3 (more informatively designated PtdIns-3,4,5-P3).
Initial characterization of PI3K activity found that it was associated with two protein subunits, one of 85 kDa (p85) and the other of 110 kDa (p110). Further work demonstrated that the p85 subunit had no intrinsic PI3K activity but that it contained a protein-protein interaction domain of the SRC homology domain 3 (SH3) type and two phosphotyrosine-binding sites of the SRC homology domain 2 (SH2) type. The presence of the SH3 domain allows p85 and p110 to form a heterodimer and the presence of the SH2 domains allows the heterodimer to bind to phosphorylated tyrosine docking sites on growth factor receptors harboring intrinsic tyrosine kinase activity such as the epidermal growth factor receptor (EGFR).
Characterization of the p110 protein demonstrated that it showed significant homology to the yeast vacuolar protein sorting-associated protein 34 (Vps34). In yeast the Vps34 protein is involved in endosomal sorting of proteins towards the vacuole which is the yeast equivalent of the mammalian lysosome. Indeed, the yeast Vps34 protein harbors PI3K activity. Additional studies demonstrated that mammalian cells also contain a PI3K activity that is not activated by receptor-mediated signaling and that this kinase has a role in vesicular trafficking.
These studies, and many others, identified several different p110 and p85 subunit genes resulting in the characterization of PI3K enzymes as a large family of enzymes. The receptor-activated PI3K members include both receptor tyrosine kinase (RTK) and G-protein coupled receptor (GPCR) activated isoforms. The GPCR activated PI3K enzymes consist of the p110 catalytic subunit and a different regulatory subunit identified as p101. The p101 subunit facilitates interaction of the catalytic p110 subunit with the βγ-subunits that are released from the heterotrimeric G-proteins activated by GPCR stimulation.
The result of this large body of work was the classification of PI3K enzymes into three distinct groups based upon structural and biochemical characteristics. The three groups are designated class I, class II, and class III. The class I and class III PI3K enzymes function as heterdimers while the class II PI3K are monomeric enzymes.
All three classes of PI3K possess a PI3K signature motif. This motif is composed of a C2 domain (a membrane targeting domain that binds Ca2+ ions), the catalytic kinase domain, and a helical domain.
The enzymes of the PI3K family harbor additional domains, distinct from the PI3K signature motif, that are critical to their function. These domains are also found in numerous other signal transduction proteins. One important domain in PI3K enzymes is the pleckstrin homology (PH) domain, a loosely conserved modular domain of about 120 amino acids. The PH domain is found in all the class I PI3K enzymes. The significance of the PH domain is that it binds to PIP2 (PtdIns-4,5-P3) as well as to PIP3 and to phosphatidylinositol-3,4-bisphosphate (PtdIns-3,4-P2). The class I PI3K also possess a RAS-binding domain (RBD) in the N-terminus that allows for stimulus-dependent RAS activation.
The regulatory subunits of the p85 family (p85, p55, and p50) class I PI3K contain two SRC homology 2 (SH2) domains, one in the N-terminus and one in the C-terminus.
Class I PI3K
Class I PI3K enzymes are receptor-regulated and Ras regulated kinases. The class I PI3K can transduce signals from both receptor tyrosine kinases (RTK) and G-protein coupled receptors (GPCR). Mammals express two subgroups of the class I PI3K isoforms, with the IA subgroup representing the RTK-activated enzymes and the IB subgroup representing the GPCR-activated enzymes.
The catalytic subunits of class I PI3K enzymes are designated p110α (encoded by the PIK3CA gene), p110β (encoded by the PIK3CB gene), p110δ (encoded by the PIK3CD gene), and p110γ (encoded by the PIK3CG gene).
The class I regulatory subunits are encoded by the PIK3R1, PIK3R2, PIK3R3, PIK3R5, and PIK3R6 genes. The PIK3R1 gene generates three splice variants that encode the p85α, p55α, and p50α proteins. The PIK3R2 gene encodes the p85β protein. The PIK3R3 gene encodes the p55γ protein. The PIK3R5 gene encodes the p101 protein. The PIK3R6 gene generates splice variants that encode p87 and p84 proteins.
Class I PI3K can generate phosphatidylinositol-3-phosphate (PtdIns-3-P), phosphatidylinositol-3,4-bisphosphate (PIP2; PtdIns-3,4-P2), and phosphatidylinositol-3,4,5-trisphosphate (PIP3; PtdIns-3,4,5-P3). The class I PI3K are the only PI3K enzymes that can generate PtdIns-3,4,5-P3.
Class II PI3K
Class II PI3K enzymes are also referred to as the PI3K-C2 kinases. Humans express three class II PI3K isoforms identified as PI3K-C2α, PI3K-C2β, and PI3K-C2γ. The PI3K-C2α enzyme is encoded by the PIK3C2A gene, the PI3K-C2β enzyme is encoded by the PIK3C2B gene, and the PI3K-C2γ enzyme is encoded by the PIK3C2G gene.
The class II PI3K are large proteins that do not form heterodimers such as is the case for the class I PI3K. The N- and C-terminal domains of the class II PI3K possess multiple interaction domains allowing for interactions with both proteins and lipids.
Class II PI3K can generate phosphatidylinositol-3-phosphate (PtdIns-3-P) and phosphatidylinositol-3,4-bisphosphate (PIP2; PtdIns-3,4-P2). The PI3K-C2α and PI3K-C2γ enzymes are the class II enzymes that are capable of generating PtdIns-3,4-P2. In certain subcellular locations evidence indicates that PI3K-C2α can also generate PtdIns-3,4,5-P3.
Class III PI3K
The class III PI3K enzyme (there is only a single class III enzyme) is the phosphatidylinositol-specific enzyme (human homolog of yeast Vps34) which is encoded by the PIK3C3 gene. The human PIK3C3 protein forms a heterodimer with a regulatory subunit that is designated p150 (Vps15 in yeast) which is encoded by the PIK3R4 gene. The p150 protein is myristoylated at the N-terminus which regulates the intracellular membrane localization of the catalytic subunit.
The major roles of the PIK3C3 encoded enzyme are in nutrient sensing, endosomal trafficking, cytokinesis, and autophagy.
Class III PI3K can generate phosphatidylinositol-3-phosphate (PtdIns-3-P).
Phosphatidylinositol 4-kinases, PI4K
The PI4K enzymes phosphorylate phosphatidylinositol (PtdIns) on the 4-OH of the inositol to generate phosphatidylinositol-4-phosphate (PtdIns-4-P). The PtdIns-4-P can then be further phosphorylated by both PI3K and phosphatidylinositol-4-phosphate 5-kinases (PIP5K) which generates PtdIns-3,4-P2 or PtdIns-4,5-P2, respectively. The PI4K do not appear to be capable of phosphorylating any other substrate besides PtdIns.
The PI4K enzymes are ubiquitously expressed and are localized to various membrane fractions that includes the plasma membrane, the lysosomes, the endoplasmic reticulum (ER), and the Golgi.
Humans express PI4K enzymes that were originally identified as type II and type III. The type II PI4K is a monomeric membrane associated kinase that has a molecular weight around 55 kDa. The type III PI4K is also membrane localized but has a molecular weight around 220 kDa. The human type II PI4K is more commonly designated PI4Kα and is encoded by the PI4KA gene. The human type III PI4K is more commonly designated PI4Kβ and is encoded by the PI4KB gene.
Phosphatidylinositol-5-Phosphate 4 Kinases (PIP4K) and Phosphatidylinositol-4-Phosphate 5 Kinases (PIP5K)
In addition to the phosphatidylinositol 3-kinases there are phosphatidylinositol kinases that phosphorylate the 4-OH and the 5-OH on inositol. Indeed, the generation of phosphatidylinositol-4,5-bisphosphate (PIP2) is the result of the concerted action of phosphatidylinositol-5-phosphate 4-kinases (PIP4K) and phosphatidylinositol-4-phosphate 5-kinases (PIP5K).
The PIP4K represent a family enzymes that are able to phosphorylate phosphatidylinositol-5-phosphate (PI5P; also designated PtdIns-5-P) on the 4-OH position of the inositol ring. Humans express three isoforms of PIP4K identified as phosphatidylinositol-5-phosphate 4-kinase type 2 alpha (PIP4Kα encoded by the PIP4K2A gene), phosphatidylinositol-5-phosphate 4-kinase type 2 beta (PIP4Kβ encoded by the PIP4K2B gene), and phosphatidylinositol-5-phosphate 4-kinase type 2 gamma (PIP4Kγ encoded by the PIP4K2C gene). The PIP4K2A encoded enzyme is found predominantly in the cytoplasm and the plasma membrane. The PIP4K2B encoded enzyme is localized in the nucleus as well as the cytosol and the plasma membrane. The specific subcellular localization of the PIP4K2C encoded enzyme has not been well defined.
The PIP5K represent a family enzymes that are able to phosphorylate phosphatidylinositol-4-phosphate (PI4P; also designated PtdIns-4-P) on the 5-OH position of the inositol ring. Humans express three isoforms of PIP5K identified as phosphatidylinositol-4-phosphate 5-kinase type 1 alpha (PIP5Kα encoded by the PIP5K1A gene), phosphatidylinositol-4-phosphate 5-kinase type 1 beta (PIP5Kβ encoded by the PIP5K1B gene), and phosphatidylinositol-4-phosphate 5-kinase type 1 gamma (PIP5Kγ encoded by the PIP5K1C gene). The enzyme encoded by the PIP5K1A gene is localized to the plasma membrane, the Golgi complex, and in nucleus. The enzyme encoded by the PIP5K1B gene is localized to the plasma
membrane and in the perinuclear region. The enzyme encoded by the PIP5K1C gene is localized to focal adhesions and adherent junctions in epithelial cells.
Nuclear Phospholipids in Signaling
The role of phosphatidylinositols (PtdIns) in signal transduction processes in the nucleus are distinct and separate from the roles of PtdIns in cytoplasmic signal transduction processes. Early evidence of nuclear-specific PtdIns functions included the observation that IGF-1 treatment of cells in culture induced decreases of phosphatidylinositol 4-phosphate (PI4P; PtdIns-4-P) and phosphatidylinositol 4,5-biphosphate (PIP2; PtdIns-4,5-P2) in the nucleus and that this was independent of the cytoplasmic counterparts. These effects were associated with increased levels of nuclear diacylglycerol (DAG) and the translocation of PKC isoforms to the nucleus.
The localization of PtdIns in the nucleus predominates in structures of interchromatin that are termed speckles. The significance of the localization of PtdIns to nuclear speckles stems from the fact that these nuclear domains are associated with chromatin remodeling and RNA splicing.
The kinases that generate the various PtdIns are described in the preceding section. One of the PIP5K enzymes (PIP5Kα) and one of the PIP4K enzymes (PIP4Kβ) are localized to the nucleus. Within the nucleus there is a higher level of phosphorylation on the 4-OH of the inositol ring compared to the 5-OH which implies that PtdIns-4-P predominates and therefore, the major pathway for the synthesis of PIP2 (PtdIns-4,5-P2) within the nucleus is via PIP5Kα. The hydrolysis of nuclear PIP2 occurs predominantly via the action of the PLCβ1 isoform, specifically the PLCβ1a splice variant.
Nuclear PIP2 (PtdIns-4,5-P2) is involved in the regulation of RNA polymerase I-mediated transcription of ribosomal RNA, the regulation of the poly(A)-polymerase involved in the transcription of stress-related mRNAs, and the targeting of proteins to splicing complexes that are involved in the export of spliced mRNAs to the cytosol.
Nuclear PIP2 (PtdIns-4,5-P2) is also involved in the regulation of cell cycle progression. The PIP5K family member, PIP5Kα, interacts with the tumor suppressor pRB which results in activation of the enzyme leading to increased production of PIP2. The hydrolysis of nuclear PIP2 by PLCβ1 correlates to cell cycle progression specifically at the G1/S and G2/M transitions since the level of PLCβ1 activity peaks at these stages. The DAG produced through the activity of PLCβ1 results in increased nuclear import of PKCα and PKCβII. These kinases phosphorylate several nuclear targets involved in cell cycle progression such as lamin B1 which is involved in nuclear envelope disassembly at mitosis.
Several genes encoding proteins involved in nuclear phosphoinositide homeostasis have been shown to be mutated in various cancers. Mono-allelic deletion of the PLCB1 gene in patients with myelodysplastic syndrome (MDS) is associated with poor prognosis. Expression of the PIP4K2A gene has been shown to relate to acute myeloid leukemia (AML) given that silencing of the gene in AML cells in culture reduces their proliferation.
Protein Kinase B: PKB/AKT
A critical regulator of cell metabolism, growth, and proliferation, that is coupled to the PI3K signaling pathway, is the Ser/Thr kinase, PKB. PKB is now more commonly identified as AKT. AKT (AK strain transforming) was originally identified as the tumor inducing gene in the AKT8 retrovirus found in the AKR strain of mice.
There are three members of the PKB/AKT family of serine/threonine kinases identified as AKT1 (PKB, also PKBα), AKT2 (PKBβ), and AKT3 (PKBγ). PKB/AKT also contains a plekstrin homology (PH) domain allowing it to be directly associated with the PI3K signal transduction pathway. Activation of PKB/AKT occurs via phosphorylation which is catalyzed by another upstream kinase which is itself activated by phosphoinositides. Specifically this PKB/AKT kinase is called phosphoinositide-dependent kinase 1, PDK1. The phosphoinositides that activate PDK1 are PIP3 generated via the PI3K enzymes.
The significance of PI3K activity in the regulation of PKB/AKT is evident from the fact that numerous PKB/AKT substrates are involved in the regulation of metabolism, cell growth, apoptosis, and autophagy. As an example, PKB/AKT phosphorylates the tumor suppressor known as tuberous sclerosis 2 (TSC2) which in turn regulates the activity of the Ser/Thr kinase mTOR (mechanistic target of rapamycin) which is in the mTOR complexes (mTORC1 and mTORC2) that control metabolism, cell growth, and autophagy. PKB/AKT also phosphorylates glycogen synthase kinase 3 (GSK3) in the insulin signaling pathway, the apoptosis promoting protein BAD (BCL-2-associated agonist of cell death), and the cell cycle regulator p21CIP. Indeed more than 100 PKB/AKT substrates have been identified.
Lysophospholipids
Lysophospholipids (LPL) are minor lipid components compared to the major membrane phospholipids such as phosphatidylcholline (PC), phosphatidylethanolamine (PE), and sphingomyelin. The LPLs were originally presumed to be simple metabolic intermediates in the de novo biosynthesis of phospholipids. However, subsequent studies demonstrated that the LPLs exhibited biological properties resembling those of extracellular growth factors or signaling molecules. The most biologically significant LPLs are lysophosphatidic acid (LPA), lysophosphatidylcholine (LPC), lysophosphatidylinositol (LPI), sphingosine 1-phosphate (S1P), and sphingosylphosphorylcholine (SPC). Each of these LPL functions via interaction with specific G-protein coupled receptors (GPCR) leading to autocrine or paracrine effects. The first LPL receptor identified was called LPA1 because it bound LPA. The first GPCR shown to bind S1P was called S1P1. For more information on the synthesis and activities of S1P go to the Sphingolipid Metabolism and Ceramides page. For more detailed information on the activities of the lysophospholipids go to the Bioactive Lipids and Lipid Sensing Receptors page.
Currently there are fifteen characterized LPL receptors. Because several of the LPL receptors were independently identified in unrelated assays, there are several different names for some members of this receptor family. In particular, there is a group of genes that were originally identified as GPCRs and called endothelial differentiation genes (EDGs) that were later found to be the same as several of the LPL receptors. Thus LPA1 is also known as EDG-2, LPA2 as EDG-4, and LPA3 as EDG-7. S1P1 is also known as EDG-1, S1P2 as EDG-5, S1P3 as EDG-3, S1P4 as EDG-6, and S1P5 as EDG-8.
Activation of the LPA receptors triggers several different downstream signaling cascades. These include activation of MAP kinase (MAPK), activation of PLC, PKB/AKT activation, calcium mobilization, release of arachidonic acid, inhibition or activation of adenylate cyclase, and activation of several small GTPases such as Ras, Rho, and Rac. The LPs exert a wide-range of biochemical and physiological responses including platelet activation, smooth muscle contraction, cell growth, and fibroblast proliferation.
LPA is produced by activated platelets, activated adipocytes, neuronal cells, as well as several other cell types. The mode(s) of LPA synthesis intracellularly remains to be fully elucidated. LPA is produced in the serum through the action of several different enzymes including monoglyceride kinase (MGL), phospholipase A1 (PLA1), secretory phospholipase A2 (sPLA2), and lysophospholipase D (lysoPLD). LysoPLD is also called autotaxin (ATX) as discussed above. Degradation of LPA occurs via lysophospholipase, lipid phosphate phosphatase, or LPA acyl transferase (also called endophilin).
S1P is stored in platelets and released upon platelet activation. Synthesis of S1P occurs exclusively from sphingosine via the action of sphingosine kinases. Degradation of S1P occurs through the action of S1P lyases or S1P phosphatases.
Lipid Phosphatases
Enzymes that dephosphorylate phospholipids belong to the subfamily of phosphatases identified as lipid phosphatases. The lipid phosphatase family of enzymes is further subdivided into four subfamilies identified as the phospholipid phosphatases, the phosphoinositide phosphatases, the sphingosine-1-phosphate phosphatases, and the lipins.
Phospholipid Phosphatases
Phospholipid phosphatases (PLPP) are also referred to as lipid phosphate phosphatases (LPP), and type 2 phosphatidic acid phosphatases. Humans express six genes in the PLPP family. The LPP are considered a subfamily of three enzymes in the larger PLPP family of phosphatases. The three LPP family members are PLPP1, PLPP2, and PLPP3 as indicated in the Table below. Humans express a seventh member of the PLPP family, PLPP7, however, the encoded protein does not possess phosphatase activity.
The enzymes encoded by the PLPP1, PLPP2, and PLPP3 genes hydrolyze a wide spectrum of lipid phosphates including phosphatidic acid (PA), lysophosphatidic acid (LPA), sphingosine 1-phosphate (S1P), ceramide 1-phosphate (C1P), and diacylglycerol pyrophosphate (DGPP). More details on the activities of LPA are presented in the Bioactive Lipids and Lipid Sensing Receptors page.
PLPP1, PLPP2, and PLPP3 are responsible for the breakdown of both intracellular and extracellular LPA and S1P, both of which are important bioactive lipids that participate in many physiological processes such as vascular development and cell cycle regulation, as well as pathological processes such as cardiovascular disease and cancer. PLPP1, PLPP2, and PLPP3 possess six transmembrane spanning domains that allow then to be localized to the plasma membrane, membranes of the endoplasmic reticulum (ER), and membranes of the Golgi.
The enzymes encoded by the PLPP4 and PLPP5 genes were originally identified as diacylglycerol pyrophosphate phosphatase-like 2 (DPPL2) and 1 (DPPL1), respectively. As the original name implies, PLPP4 and PLPP5 prefer diacylglycerol pyrophosphate (DGPP) as a substrate but they also hydrolyze phosphatidic acid (PA) and lysophosphatidic acid (LPA).
The enzyme encoded by the PLPP6 gene was originally identified as polyisoprenyl diphosphate phosphatase 1 (PDP1) and also as candidate sphingomyelin synthase type 2 (CSS2). PLPP6 exhibits highest affinity for presqualene diphosphate (PSDP), but will also hydrolyze farnesyl diphosphate (FDP), S1P, LPA, and PA.
Table of Human Phospholipid Phosphatases (PLPP)
Symbol | Enzyme Name | Other Common Abbreviations |
PLPP1 | phospholipid phosphatase 1 | PPAP2A, LPP1 |
PLPP2 | phospholipid phosphatase 2 | PPAP2C, LPP2 |
PLPP3 | phospholipid phosphatase 3 | PPAP2B, LPP3 |
PLPP4 | phospholipid phosphatase 4 | PPAPDC1, PPAPDC1A, DPPL2 |
PLPP5 | phospholipid phosphatase 5 | PPAPDC1B |
PLPP6 | phospholipid phosphatase 6 | PPAPDC2 |
Lipins
In addition to the PLPP family of phospholipid phosphatases, several of which hydrolyze phosphatidic acid to DAG, there are the enzymes of the lipin (LPIN) family that also are responsible for the hydrolysis of phosphatidic acid. Humans express three genes in the LPIN family, LPIN1, LPIN2, and LPIN3. The LPIN1 encoded enzyme is the most well characterized with three identified isoforms, lipin-1α, lipin-1β, and lipin-1γ. The major function of the lipin family of phosphatidic acid phosphatases is the generation of DAG from phosphatidic acid that can then be used in the synthesis of various membrane-associated phospholipids such as phosphatidylethanolamine (PE), phosphatidylcholine (PC), and phosphatidylserine (PC). The lipin family enzymes are involved in membrane phospholipid homeostasis in both the cytosol and the nucleus. Indeed, these enzymes possess a nuclear localization signal that targets them to this organelle.
Phosphoinositide Phosphatases
Polyphosphoinositides represent an important family of signaling molecules with the most well characterized being phosphatidylinositol-4,5-bisphosphate, PIP2 (PtdIns-4,5-P2). Polyphosphoinositide homeostasis represents a balance between synthesis via the actions of various kinases and turnover via the actions of various phosphoinositide phosphatases. Humans express a large family of genes that encode enzymes of the phosphoinositide phosphatase family, many of which are described in the following Table.
Numerous growth factors, including insulin, activate signaling cascades upon binding to their cell surface receptors leading to the activation of the enzymes of the phosphatidylinositol 3-kinase (PI3K) family. Some of the PI3K family enzymes phosphorylate PIP2 generating phosphatidylinositol-3,4,5-trisphosphate (PIP3; also designated PtdIns-3,4,5-P3). In order to regulate the level of signal intensity PIP3 is dephosphorylated by PTEN, or members of the polyphosphate 5-phosphatase subfamily of phosphoinositide phosphatases, regenerating PIP2.
Table of Human Phosphoinositide Phosphatases
Symbol | Enzyme Name | Functions / Comments |
BPNT1 | 3′(2′), 5′-bisphosphate nucleotidase 1 | predominant activity is the conversion of adenosine 3′-phosphate 5′-phosphosulfate (PAPS) to adenosine 5′-phosphosulfate (APS) and 3′(2′)-phosphoadenosine 5′- phosphate (PAP) to AMP; although classified as a member of the phosphoinositide phosphatase family it only exhibits weak affinity for dephosphorylation of inositol 1,4-bisphosphate (Ins-1,4-P2) and inositol 1,3,4-trisphosphate (Ins-1,3,4-P3) |
FIG4 | FIG4 phosphoinositide 5-phosphatase | is an inositol polyphosphate-5-phosphatase; primary substrate is phosphatidylinositol-3,5-bisphosphate (PtdIns-3,5-P2); mutations lead to two forms of nervous system lysosomal storage disease; one form occurs in spinal sensory neurons and the second form occurs in cortical/spinal motor neurons and glia; deficiency in FIG4 is commonly known as Charcot-Marie-Tooth disease type 4J (CMT4J); patients with CMT4J present with clinical and pathophysiological phenotypes indicative of spinal motor neuron degeneration and segmental demyelination |
IMPA1 | inositol monophosphatase 1 | yields myo-inositol from various myo-inositol monophosphates; is likely to be a physiological target for the function of lithium in the treatment of bipolar disorder |
IMPA2 | inositol monophosphatase 2 | yields myo-inositol from various myo-inositol monophosphates; polymorphisms in the promoter region of the IMPA2 gene have been found to be associated with bipolar disorder |
INPP1 | inositol polyphosphate-1-phosphatase | hydrolyzes the phosphate from the 1-position of phosphatidylinositol 1,4-bisphosphate (PtdIns-1,4-P2) and phosphatidylinositol 1,3,4-trisphophosphate (PtdIns-1,3,4-P3) |
INPPL1 | inositol polyphosphate phosphatase like-1 | more commonly known as SHIP2 [SH2 (Src homology 2)-domain containing inositol phosphatase 2]; hydrolyzes the 5-phosphate of phosphatidylinositol-3,4,5-trisphosphate (PtdIns-3,4,5-P3) to produce PtdIns-3,4-P2; negatively regulates insulin signaling and glucose homeostasis; polymorphisms are associated with a predisposition to insulin resistance |
INPP4A | inositol polyphosphate-4-phosphatase type II A | is classified as a member of the C2 domain-containing superfamily which also includes the PI3K family; dephosphorylates phosphatidylinositol-3,4-bisphosphate (another form of PIP2 more correctly designated PtdIns-3,4-P2) to phosphatidylinositol-3-phosphate |
INPP4B | inositol polyphosphate-4-phosphatase type II B | is also considered a member of the C2 domain containing superfamily; dephosphorylates phosphatidylinositol-3,4-bisphosphate (another form of PIP2 more correctly designated PtdIns-3,4-P2) to phosphatidylinositol-3-phosphate |
INPP5A | inositol polyphosphate-5-phosphatase A | hydrolyzes the phosphate from the 5-position of phosphatidylinositol-1,4,5-trisphosphate (PtdIns-1,4,5-P3: commonly just IP3); the action of this phosphatase restricts the level of calcium (Ca2+) released from the endoplasmic or sarcoplasmic reticulum (ER/SR) via the action of IP3 binding to its ER/SR localized receptor |
INPP5B | inositol polyphosphate-5-phosphatase B | hydrolyzes the phosphate from the 5-position of phosphatidylinositol-4,5-bisphosphate (PtdIns-4,5-P2) and PtdIns-3,4,5-P3 |
INPP5D | inositol polyphosphate-5-phosphatase D | more commonly known as SHIP1 [SH2 (Src homology 2)-domain containing inositol phosphatase 1]; primarily responsible for the hydrolysis of the 5-phosphate of phosphatidylinositol-3,4,5-trisphosphate (PtdIns-3,4,5-P3) to produce PtdIns-3,4-P2; can also hydrolyze the 5-phosphate of phosphatidylinositol-4,5-bisphosphate (PtdIns-4,5-P2) and inositol 1,3,4,5-tetrakisphosphate (Ins-1,3,4,5-P4) |
INPP5E | inositol polyphosphate-5-phosphatase E | hydrolyzes the phosphate from the 5-position of phosphatidylinositol-1,4,5-trisphosphate (PtdIns-1,4,5-P3: commonly just IP3); the action of this phosphatase restricts the level of calcium (Ca2+) released from the endoplasmic or sarcoplasmic reticulum (ER/SR) via the action of IP3 binding to its ER/SR localized receptor; may also hydrolyze PtdIns-3,4,5-P3 |
INPP5F | inositol polyphosphate-5-phosphatase F | also known as SAC2 (Sac domain-containing phosphoinositide 4-phosphatase 2); the SAC (suppressor of actin) domain was originally identified in the yeast Sac1 gene and synaptojanin 1 (SYNJ1) and is the domain that harbors the phosphatidylinositol polyphosphate phosphatase activity |
INPP5J | inositol polyphosphate-5-phosphatase J | most likely dephosphorylates the 5-position of phosphatidylinositol-3,4,5-trisphosphate (PtdIns-3,4,5-P3) and PtdIns-4,5-P2 |
INPP5K | inositol polyphosphate-5-phosphatase K | more commonly known as SKIP (skeletal muscle- and kidney-enriched inositol phosphatase); negatively regulates insulin signaling and glucose homeostasis |
MINPP1 | multiple inositol-polyphosphate phosphatase 1 | hydrolyzes the phosphate from the 3-position of various phosphatidylinositols; is the only phospholipid phosphatase that can dephosphorylate inositol pentakisphosphate (InsP5) and inositol hexakisphosphate (InsP6); also converts 2,3 bisphosphoglycerate (2,3-BPG) to 2-phosphoglycerate; this latter activity was originally thought to be exclusive to bi-functional enzyme 2,3-bisphosphoglycerate mutase (BPGM) in the Rapoport-Luebering shunt of the glycolytic pathway |
MTM1 | myotubularin 1 | dephosphorylates phosphatidylinositol 3-monophosphate (PtdIns-3-P) and phosphatidylinositol-3,5-bisphosphate (PtdIns-3,5-P2); has also been shown to dephosphorylate proteins phosphorylated on Ser and Tyr residues |
MTMR1 | myotubularin related protein 1 | hydrolyzes the phosphate from the 3-position; near exclusive activity for dephosphorylation of phosphatidylinositol 3-phosphate (PtdIns-3-P) |
MTMR2 | myotubularin related protein 2 | hydrolyzes the phosphate from the 3-position of phosphatidylinositol 3-phosphate and phosphatidylinositol 3,5-bisphosphate (PtdIns-3,5-P2) |
MTMR3 | myotubularin related protein 3 | hydrolyzes the phosphate from the 3-position of phosphatidylinositol 3-phosphate and phosphatidylinositol 3,5-bisphosphate (PtdIns-3,5-P2); may also dephosphorylate proteins phosphorylated on Ser, Thr, and Tyr residues |
MTMR4 | myotubularin related protein 4 | |
MTMR6 | myotubularin related protein 6 | hydrolyzes the phosphate from the 3-position of phosphatidylinositol 3-phosphate and phosphatidylinositol 3,5-bisphosphate (PtdIns-3,5-P2) |
MTMR7 | myotubularin related protein 7 | hydrolyzes the phosphate from the 3-position of phosphatidylinositol 3-phosphate and inositol 1,3-bisphosphate (Ins-1,3-P2) |
MTMR8 | myotubularin related protein 8 | hydrolyzes the phosphate from the 3-position of phosphatidylinositol 3-phosphate and phosphatidylinositol 3,5-bisphosphate (PtdIns-3,5-P2) |
MTMR14 | myotubularin related protein 14 | hydrolyzes the phosphate from the 3-position of phosphatidylinositol 3-phosphate and phosphatidylinositol 3,5-bisphosphate (PtdIns-3,5-P2) |
OCRL | OCRL inositol polyphosphate-5-phosphatase | hydrolyzes phosphatidylinositol-4,5-bisphosphate (PtdIns-4,5-P2) and PtdIns-3,4,5-P3 |
PIP4P1 | phosphatidylinositol-4,5-bisphosphate 4-phosphatase 1 | hydrolyzes phosphatidylinositol-4,5-bisphosphate (PtdIns-4,5-P2) to phosphatidylinositol-4-phosphate (PtdIns-4-P) |
PIP4P2 | phosphatidylinositol-4,5-bisphosphate 4-phosphatase 2 | hydrolyzes phosphatidylinositol-4,5-bisphosphate (PtdIns-4,5-P2) to phosphatidylinositol-4-phosphate (PtdIns-4-P) |
PTEN | phosphatase and tensin homolog deleted on chromosome 10 | is a phosphatidylinositol-3-phosphate phosphatase; originally identified as a tumor suppressor located on chromosome 10q23; was originally thought to be a protein tyrosine phosphatase (PTP) due to the presence of a canonical HCxxGxxR motif typical of PTP enzymes; the Cys (C) residue in this motif is critical for catalytic activity and the Arg (R) residue binds the phosphoryl group of the substrate; dephosphorylates phosphatidylinositol-3-phosphate (PtdIns-3-P), PtdIns-3,4-P2, and PtdIns- 3,4,5-P3, |
PTPMT1 | protein tyrosine phosphatase mitochondrial 1 | dephosphorylates phosphatidylglycerophosphate (PGP) to phosphatidylglycerol (PG); PGP is an intermediate in the synthesis of cardiolipins which are a family of mitochondria-specific phospholipids |
SACM1L | SAC1 like phosphatidylinositide phosphatase | dephosphorylates multiple phosphoinositides, such as phosphatidylinositol-3-phosphate (PtdIns-3-P), PtdIns-4-P, PtdIns-5-P and PtdIns-3,5-P2 to form phosphatidylinositol (PtdIns); the SAC (suppressor of actin) domain was originally identified in the yeast Sac1 gene and synaptojanin 1 (SYNJ1) and is the domain that harbors the phosphatidylinositol polyphosphate phosphatase activity |
SYNJ1 | synaptojanin 1 | SAC domain-containing phosphatase; the SAC (suppressor of actin) domain was originally identified in the yeast Sac1 gene and SYNJ1 and is the domain that harbors the phosphatidylinositol polyphosphate phosphatase activity; hydrolyzes PtdIns-4,5-P2, PtdIns-3,4,5-P3, Ins-1,4,5-P3, and Ins-1,3,4,5-P4; primarily expressed at postsynaptic nerve terminals in the brain |
SYNJ2 | synaptojanin 2 | SAC domain-containing phosphatase; the SAC (suppressor of actin) domain was originally identified in the yeast Sac1 gene and SYNJ1 and is the domain that harbors the phosphatidylinositol polyphosphate phosphatase activity; hydrolyzes PtdIns-4,5-P2, PtdIns-3,4,5-P3, Ins-1,4,5-P3, and Ins-1,3,4,5-P4; |
Although there are several myotubularin-related (MTMR) protein encoding genes that are not listed in the Table above, these proteins function not as phosphatases but as accessory (adaptor) proteins to the phosphatases of the myotubularin-related family. These accessory MTMR proteins are encoded by the MTMR9, MTMR10, MTMR11, and MTMR12, genes. The SBF1 (SET binding factor 1) gene has also been identified as MTMR5. The SBF2 (SET binding factor 2) gene has also been identified as MTMR13.